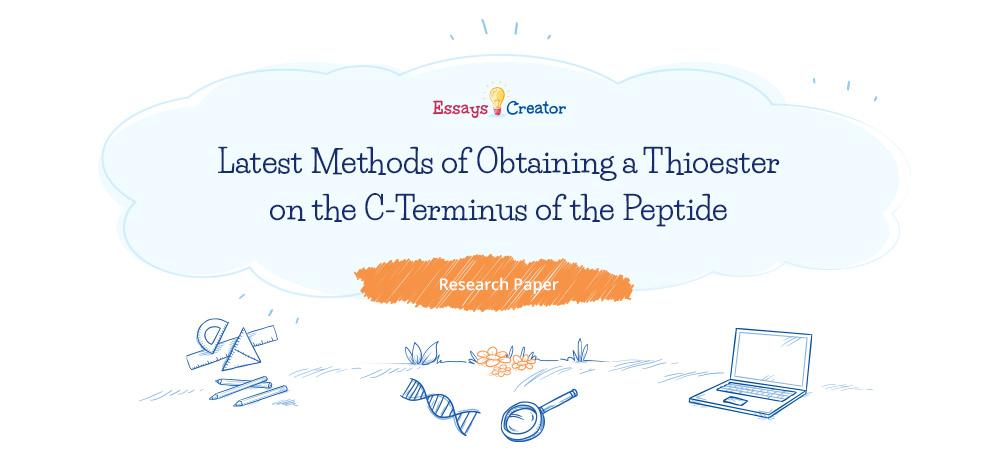
The formation of peptides in the body takes few minutes.
Chemical synthesis in the laboratory is a lengthy process that can take several days, and the development of technology of synthesis can last for several years. However, despite this, there are some compelling arguments in favor of the work on the synthesis of analogs of natural peptides. First, by chemical modification of peptides, the hypothesis of the primary structure may be supported. Amino acid sequences of some hormones become known due to the synthesis of their counterparts in the laboratory.
Second, synthetic peptides can further explore the relationship between the structure of the amino acid sequence and its activity.
To clarify the relationship between the concrete structure of the peptide and its biological activity a lot of research was done on the synthesis of thousands of analogs. As a result, it was found out that the replacement of a single amino acid in the structure of the peptide is capable to increase its biological activity several times or change its direction. A change in length of the amino acid sequence helps determine the location of active sites and the site of peptide receptor interaction.
Order your Research Paper help today!
Third, due to the modification of the original amino acid sequence, it is possible to obtain pharmacological preparations.
Analogs of natural peptides reveal a more efficient configuration of the molecules, which increases the biological effect or makes it longer.
Fourth, the chemical synthesis of peptides is economically beneficial.
Most therapeutic drugs would cost ten times more if they were made based on a natural product.
Often active peptides are found in nature only in nanogram quantities. Moreover, cleaning methods and isolation of peptides from natural sources cannot completely separate the desired amino acid sequence of the peptides of the opposite or other action. In the case of specific peptides synthesized by the human body, one can only get them by the synthesis in the laboratory.
Fundamentals of peptide synthesis to date can be attributed to a textbook. Real practical significance, they no longer have to force the rapid development in the last 10-15 years, methods for producing peptides and proteins by genetic engineering.
In 1900, German organic chemist Hermann Emil Fischer hypothesized that peptides are composed of chains of amino acids formed by certain constraints. In 1902, he received overwhelming evidence about the existence of a peptide bond. In 1905 he developed a general method by which it became possible to synthesize peptides in vitro.
Gradually, scientists studied the structure of various compounds, developed methods for the separation of polymer molecules monomers, and synthesized more and more peptides. Currently, there are more than 1500 kinds of peptides identified with their particular properties and methods of synthesis.
Peptide synthesis is reduced to the formation of an amide bond between α-carboxyl and α-amino groups of two amino acids. During the reaction, water molecule splits and carboxyl or amino group must be activated for its implementation. To date, there are different ways of activation, but few of them are widely applied.
How it works
Step 1
Visit our website and go to the order formStep 2
Fill in specific essay details in your order description sectionStep 3
Pay for your custom essay and get your order verifiedStep 4
Process of writing your academic assignmentStep 5
Editing and anti-plagiarism checkStep 6
On-time delivery of an already written essayFor unambiguous peptide synthesis in a mixture of amino acids, it is not enough to activate their functional groups. The reason is that activated amino acid molecules can react with molecules both with a similar and different structure than other amino acids (Blanco-Canosa and Dawson 2011):
For this reason, the synthesis of peptides requires the protection of all functional groups, whose participation in the reaction is not desirable. Used in peptide synthesis, protecting groups are divided into amino-(or N)-protective (X) and carboxyl (or C) protection (Y).
In addition to α-amino and α-carboxyl, groups also block functional groups in R-residue (they all share the common name – ω-group) if the latter can cause adverse reactions.
Protecting groups in peptide synthesis regardless of whether they are blocking X-, Y-, or ω-group must meet the following requirements:
- They must be available or easily synthesized with good quantitative yields;
- They should apply to as many methods of peptide synthesis;
- They are not supposed to have side effects or facilitate the flow of such;
- They must be easily and selectively removed.
In practice, all these conditions are rarely met. Therefore, it is necessary to stop at some compromise solution.
In general terms, the process of peptide synthesis can be expressed by the following scheme:
In general, peptide synthesis involves the following stages: protection of amino components carboxy, amino carboxycomponents protection, protection of carboxy-and amino side-chains; carboxy groups carboxycomponents activation, synthesis, peptide bond formation, removal (removal) of protecting groups, and selection of peptide.
Interestingly, different authors (and manufacturers too) so far did not agree to assume the same C-peptide. There are three versions of C-peptide. The first version (the longest) of the sequence is different from insulin, proinsulin, that is, C-peptide flanked on two sides by cation pairs. Second – C-peptide with a cationic pair on C-terminus (the product of the proinsulin proteolysis with trypsin), and the third (the shortest) C-peptide without cation pairs.
Latest studies (Chan and White 2000) show that C-peptide should be the last option because of cation pair, which is cleaved from the C-terminus of the B-chain of insulin during ripening hormone, which is similar to the cation pair in the C-terminal and C-peptide also recognized by enzymes (e.g. carboxypeptidase B). Consequently, the “third option” is formed in the biosynthesis of C-peptide.
With this method of genetic engineering, C-peptide and insulin are produced in the course of enzymatic digestion with trypsin and carboxypeptidase B proinsulin, which in turn is derived from fusion protein released from damaged cells of recombinant strains of E. coli.
Russian scientists Akimov and Maltsev used strain JM 109 N1864 (State Collection VNIIA) with built-in nucleotide sequence plasmid expressing fusion protein, which consists of linear proinsulin and is attached to the N-terminal methionine residue in protein fragment Staphylococcus aureus (Mackin 1999). Rich biomass cultivation of recombinant cells provides the start of production of hybrid proteins isolated and sequential transformation that results in final products (C-peptide and insulin).
Another group of researchers in a bacterial expression system obtained a recombinant fusion protein consisting of human proinsulin and driven by a methionine residue polyhistidine “tail”. It was isolated using chelate chromatography on Ni-agarose (Ford, Suominen, and Glatz 1991) from inclusion bodies and was digested with CNBr. The authors found that the isolated protein is S-sulfonated. Chart-making and mass spectrometric analysis of the resulting proinsulin was purified by ion-exchange chromatography on the anion and HPLC. It showed the presence of disulfide bridges, corresponding to a disulfide bridge of native human proinsulin. Later, in the work of Cowley and Mackin (1997), it was described by a simplified procedure for separating proinsulin. This produced a similar pattern with only HPLC.
In “Generation of Human Insulin” (Generation of Human Insulin 1996), the elaboration of a new improved method for obtaining human insulin by genetic engineering in prokaryotic cells was described. The authors found that insulin produced in their structure and biological activity identical hormone secretions from the pancreas. Recently, attention has been given to simplifying the procedure for the production of recombinant insulin by genetic engineering. So Castellanos-Serra (1996) obtained a fusion protein composed of IL-2 leader peptide attached to the N-terminus of proinsulin, a lysine residue. Protein is efficiently expressed and localized in inclusion bodies. Once isolated, the protein was cleaved with trypsin to produce insulin and C-peptide. Another research by Nilsson (1996) acted the same way. The fusion protein of proinsulin and two synthetic binding domain IgG derived from staphylococcal protein A was located in inclusion bodies but had a higher level of expression. Protein was isolated by affinity chromatography using IgG and treated with trypsin and carboxypeptidase B. The resulting insulin and C-peptide were purified by HPLC.
In another work by Sun, Tang, and Hu (1995) the production of mutant proinsulin was described, which included replacing Arg32Tyr. When coupled with cleavage of the protein with trypsin and carboxypeptidase B, they formed native insulin and C-peptide containing a tyrosine residue. Last, 125I labeling is widely used in radioimmunoassay. Polypeptides intended for the manufacture of medicinal products must be of high purity.
Therefore, high-performance control of the purity of products is ensured at each stage of production. Earlier by RP HPLC and IO were characterized proinsulin-S-sulfonate, proinsulin, separate A-and B-chain and S-sulfonate (Peter eds. 1997), and special attention was paid to the fluorescent derivative of insulin. Some authors have investigated the applicability of information content and chromatographic methods in the analysis of products of all stages of production of human insulin and made regulations chromatographic operations to effectively separate and characterize resulting products. Divided insulin derivatives with the use of bifunctional sorbents (hydrophobic and ion exchange HPLC), the possibility of controlling the selectivity of separation by varying the contribution of each interaction, thus achieving greater efficiency in the separation of close analogs of protein. In addition, an approach to automate and accelerate the processes of determining the purity and quantity of end products was developed.
When creating fusions, the ratio of molecular weight of the protein carrier and the target polypeptide is very important. Therefore, in the work of Jonasson and his colleagues (1998) the construction of fusions is described, where the carrier protein used binding protein human serum albumin, to which one, three, and seven C-peptide with tyrosine residues in the N-terminus was attached. C-peptide is joined on a “head to tail” with the amino acid spacer bearing Sfi I restriction site and two arginines at the beginning and the end of the spacer for the subsequent cleavage of the fusion protein with trypsin. Fusion proteins were localized predominantly in the cytoplasm and had similar levels of accumulation (about 50 mg per liter of overnight culture). HPLC cleavage products of three fusion proteins showed that cleavage of the C-peptide is quantified. This method allows the use of multimeric synthetic genes for target polypeptides on an industrial scale.
The same group of authors (Jonasson eds. 2000) obtained a highly strain-producing fusion protein containing incorporates seven C-peptide based on strain Escherichia coli O17. The level of accumulation of fusion protein in the cytoplasm was 1.8 grams per liter of an overnight culture. The developed technology of C-peptide included precipitation of nucleic acids after cell lysis, followed by isolation of fusion protein by ion-exchange chromatography on Q Sepharose FF (anion). Then, the purified fusion protein was subjected to proteolysis with trypsin, and cleavage products were separated with HPLC and gel filtration. This method allowed us to obtain 400 mg of C-peptide (purity 95%).
Despite the variety of methods for C-peptide of human proinsulin, they all have some drawbacks. Thus, a group of methods for C-peptide with insulin based on a microbiological synthesis of polypeptide structures (bearing in its composition proinsulin and an affine part), which are located in inclusion bodies in the insoluble form. Isolation of these proteins leads to several technical problems associated with the transfer of such polypeptides in a soluble state. To assist in the selection of C-peptide it is advisable to create high-producing strains of proteins (bearing in the structure of human proinsulin ), which would be placed in cells in a soluble form. One solution to this problem would be to create a genetically engineered structure based on carrier proteins (such as streptavidin), the products of which are expressed in the periplasmic space of cells in a soluble form.
In addition, in terms of technical efficiency, methods of preparation of C-peptide with insulin are disadvantageous because of the low yield of the desired peptide (C-peptide). A significant increase in the yield of the desired peptide can be obtained by methods of individual C-peptide in fusion proteins. When creating such structures, it is appropriate to use the principle of multimeric target peptide (Jonasson eds. 1998, 2000) i. e. recombinant constructs in which affine components connected in series for several C-peptide monomers on a “head-to-tail”. In addition, the amino acid sequence of C-peptide (the last two C-terminal amino acids Lys-Arg is cationic couple learned trypsin) allows such construction without the use of amino acid spacers between C-peptide.
Another advantage of the method for the C-peptide as a genetically engineered fusion design to a carrier protein (compared with the methods of preparation of C-peptide with insulin) is the lack of technological cycle stages of processing products of proteolysis with trypsin carboxypeptidase B, which is a quite expensive enzyme.
Therefore, there are all prerequisites for obtaining microbial C-peptide is not only with insulin, but also individually. However, most companies are still selling products of C-peptide, obtained by chemical synthesis. This situation may be due to the disadvantages of obtaining microbial C-peptide.
Solid-phase synthesis of peptides was proposed by Merrifield of Rockefeller University (Nobel Prize 1984). This method is based on the assembly of the peptide on insoluble polymer-supported sequential addition of amino acid residues with protected α-amino and side groups. Polymeric resin based on 2-hlortritilhlorida, which was first proposed to be used by K. Barlos, is not resistant to acids (Jones 2007). The spatial structure of the polymer resin as well as moderate acidity needed to break ties in chains make resin useful for many applications. For N-α-amino protecting group Fmoc-use is not resistant to the action of bases. After removal of the protective group of the following protected amino acid is added with a binding agent or pre-activated protected amino acid derivative.
The final peptide is attached to the polymer matrix through its C-terminal group. The bond to the polymer can be cleaved by getting peptidoacid or amide (depending on the used binding agent). Often, to protect side-chains of selected groups such splitting is carried out simultaneously with the separation of the peptide from the polymer matrix.
One of the areas of solid-phase synthesis in the synthesis of peptide chains contains histidine and serine. Its essence is as follows (1): a method of solid-phase peptide synthesis, including the addition of N-terminal amino acids protected to an inert solid support, followed by the addition of N-protected amino acid to the peptide chain is scalable, with the side-chain of the serine residue temporary protecting group labile towards agents used to remove alpha-amino protecting groups, and histidine side-chain, if present, protect the group labile to the alpha aminodeblocking agent; removal of the protecting group at the conclusion, cleavage of the polypeptide carrier using aminolysis or ammonolysis by issuing of the compound formed:
R1-R2-R3-Ser-Tyr-R4-heu-R5-Pro-R6, (1)
where R1 -pyro -Glu, N-AcDNal; R2His, DpClPhe, DpFPho, R3= ???, ?-???; R4= DNal(2), Dh Arg(Et)2 Dh ???(Bu), Dh Arg(CH2CF3)2 R5= ???, L-Arg(Et)2 L-h???(Bu), L-Harga(CH2CF3)2 R6 GlyNH2 DAla NH2
The invention relates to a solid phase synthesis of analogs releasing hormone luteinizing hormone (LN-releasing hormone) by the method of minimal protection.
The scheme of solid-phase peptide synthesis by Merrifield is presented as follows in Figure 1:
LH-RH analogs can be synthesized by various methods such as those described by Stuart and Yang and Meynenhofer, Shredder, and Lyubke (Chan and White 2000). Methods can be broadly characterized as either solution-phase or solid-like techniques. Both methods include the sequential addition of amino acids to a growing peptide chain. Normally, either the amino or carboxyl group of the first amino acids protects through the suitable protecting group. The protected amino acid can then be either attached to an inert solid support or can be used in the solution by adding the following protected amino acid sequence in the conditions suitable for the formation of an amide bond. The protecting group is then split again from the added amino acid residue followed by the addition of the next amino acid, and so on. Once all required amino acids are attached in the proper sequence, any remaining protecting groups and remove any solid support to give the final polypeptide. A simple modification of this general technique can add more than one amino acid at a time to a growing chain, for example, by coupling a protected tripeptide with a protected dipeptide that provides a pentapeptide.
Stricter conditions for solid-phase synthesis usually require that any reactive side-chains of amino acids were protected during the formation of an amide bond. Side-chain protecting groups are usually split off in a separate step after cleavage of polypeptide formed from an inert carrier, or in conjunction with this cleavage.
Understanding the benefits and efficiency of solid-phase peptide synthesis in a continuous process, when reactants are continuously circulated through a packed bed peptidopolimera came in the late 1970s. However, the first attempt to direct this approach brought more disappointment and questioned the fundamental compatibility of gel polymers, swelling volume of which can fluctuate significantly, and conventional reactors column type, the amount of which is rigidly fixed. As a consequence, there are voids in the reactor or unacceptably high pressure in the flow (70 atm or more) because of compaction of swelling of the polymer, which in the aggregate destroys all benefits of a flow-through mode urgency of radical solutions to the problem. The nature of gel polymer has multiplied with the rapid development of solid-phase combinatorial chemistry, but all solutions offered by the scientists are a compromise and have one thing in common: they are based on masking or suppressing the elevon nature of the polymer reagent to improve its compatibility with standard flow reactor.
Another problem of modern solid-phase peptide synthesis is the lack of universal non-invasive monitoring techniques that can recognize difficult sections of the polypeptide chain assembly in real-time, regardless of chemical methodology. Availability of such methods, which give the basis for timely adjustment and optimization of the process depends critically on the reliability and result of automatic solid-phase synthesis. Key achievements in this area show synthesizers MilliGen 9050 +, ABI 432a, ABI 433A that implement feedback control in combination with spectrophotometric and/or conductivity monitoring. However, the range of applicability of both methods is limited by Fmoc-chemistry. Against this background, a more economical and better Boc methodology is developed at a disadvantage, which determines the development of high relevance of Sun-compatible methods of monitoring in general and automated non-invasive approaches in particular.
Free Extras:
- FREE revision (within 2 days)
- FREE title page
- FREE bibliography
- FREE outline (on request)
- FREE e-mail delivery
- FREE formating
We Guarantee:
- Quality research and writing
- 24/7/365 Live support
- MA, BA, and PhD degree writers
- 100% Confidentiality
- No hidden charges
- Never resold works
- 100% Authenticity
Paper Format:
- 12 pt. Times New Roman
- Double-spaced/Single-spaced papers
- MA, BA, and PhD degree writers
- 1 inch margins
- Any citation style
- Up-to-date sources only
- Fully referenced papers